
Flexible Optical Brain-Machine
Interfaces & Wearable Biosensors
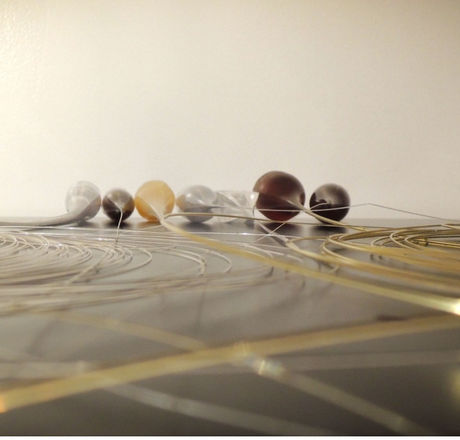
Recent advances in bioelectronics help human not only understand the mechanisms of complicate biological/neurological system but also enable the treatment of related disease. However, practical use of the devices is still limited due to the lack of the flexibility and supposedly caused immune responses. Moreover, integration of diverse modalities within a single biocompatible platform still remains a challenge. Using thermal drawing process, which is conventionally used for the fabrication of optical fibers, we produced miniature and flexible probes with optical (optogenetics), electrical (electrophysiological recording), and microfluidic (drug/virus delivery) capabilities all within the dimensions of few tens to hundreds micrometers. These organic materials-based devices allowed to deliver and receive a multitude of neuronal signals not only for brain mapping but also spinal cord modulation in freely moving animals. With this system, we are developing advanced platforms of the fiber-based devices which enables targeting of biological/neural systems in various positions of our bodies. With developments of various materials, structures, and functionalities for the fibers, we will apply them in bio-applications from bio-sensor/actuator to bioelectronic medicine.
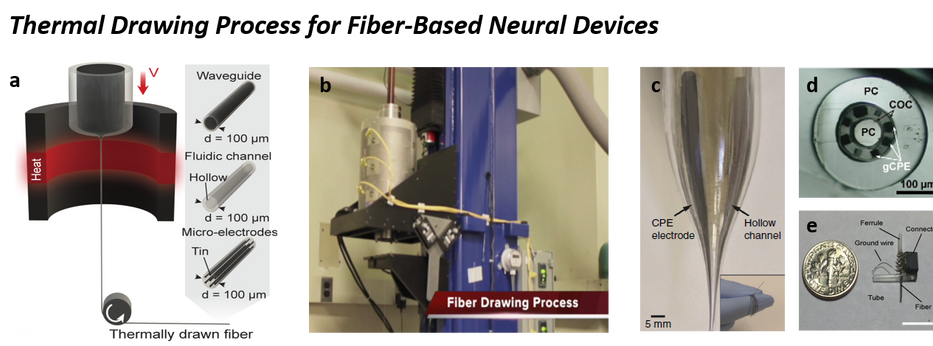
Nature Neuroscience (2017)
Nature Communications (2021)
Design and Fabrication of Multifunctional Fiber-based Neural Devices. (a) Illustration about thermal drawing process (TDP) for the fabrication of multifunctional fiber. Optical waveguide, fluidic channel, and micro-electrodes can be drawn individually by choosing proper material systems. (b) Picture of fiber drawing tower. The diameter of the resulting fiber is determined by the ratio of the capstan and feed speeds and is monitored continuously during the process by a laser sensor. (c) Photograph of preform and a bundle of fiber after the fiber drawing. (d) Cross-sectional microscope image of the multimodal fiber with optical waveguide (PC/COC), electrodes (CPE) and microfluidic channels. (e) Picture of a fiber devices outfitted with an optical ferrule, electrical connector and an injection tube. The weight of the device varied between 0.3–0.5 g. Scale bar represents 10 mm.

Science Advances (2017)
Nature Electronics (2021)
Neural Applications for Optical Neural Interfaces. (a) Mice implanted with a multifunctional neural devices into their brain and spinal cords. (b) Electrophysiological recordings during optical stimulation in the medial prefrontal cortex (mPFC) using a fiber probe between 2 and 21 d after transfection with AAV5-CaMKIIa::ChR2-eYFP. There are potential changes corresponding to the optical stimulation from 11 days after virus injection. (c) Velocity and representative trajectories recorded for mice implanted with fiber probes injected with AAV5-CaMKIIa::ChR2-eYFP (or control virus AAV5-CaMKIIa::eYFP) in mPFC during open field test (OFF/ON/OFF optical stimulation). Velocity increases with optical stimulation.

Advanced Materials (2017)
Fabrication of holographic hydrogel bio-sensor for glucose monitoring. (a) Coupling the p(AM‐co‐PEGDA) hydrogel fibers with fiber optic cables. Scale bar = 5 mm. The inset shows the optical fiber coupled to p(AM‐co‐PEGDA) fiber (b) The integration of hydrogel fibers with needles for creating insertable devices. (c) Hydrogel fibers in hypodermic needles at different light intensities. (d) The implantation of hydrogel optical fibers in porcine tissue. Scale bar = 3 mm. (e) Wavelength shift of the holographic film resulting in color change and quantification of glucose level from it.